By Lance C. Dalleck, Ph.D.
Recovery from exercise is a vital component of the overall training program, and paramount for performance and continued improvement. If the rate of recovery is appropriate, higher training volumes and intensities are possible without the detrimental effects of overtraining. In the August 2012 issue of ACE Certified News, we explored the physiological mechanisms underpinning recovery. We also discussed the various components of the exercise program (i.e., the FITT) and how these features can be modified to optimize recovery. In this article, we turn our focus to the nutritional aspect of recovery by highlighting six evidence-based nutritional strategies that your clients can use to safely and effectively facilitate recovery following intense workouts.
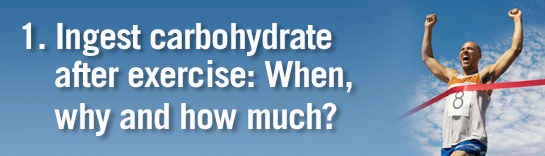
Ingesting some form of carbohydrate after exercise is a classic nutritional recommendation for recreational enthusiasts and athletes alike. Following prolonged and exhaustive endurance-related exercise, the most important factor determining the timeframe to recovery is muscle glycogen replenishment (Ivy, 2004). While it is well established that postexercise carbohydrate (CHO) ingestion is critical to the synthesis of muscle glycogen, only recently have we begun to better understand the precise timing of CHO ingestion and optimal CHO dosage (Beelen et al., 2010).
Postexercise muscle glycogen replenishment occurs in two phases: A rapid rate that persists for 30 to 60 minutes after exercise cessation and a considerably reduced rate (60–90%) in the time period afterward.
Muscle glycogen replenishment requires the entry of blood glucose into the muscle cell. This process is facilitated when GLUT-4 transporter proteins migrate from the cell cytoplasm and bind to the cell membrane. Both skeletal muscle contraction and insulin serve as signals for GLUT-4 transporter proteins to bind to the cell membrane and facilitate glucose uptake. The initial rapid rate of glucose flux into the muscle cell for glycogen resynthesis is mediated by skeletal muscle contraction–stimulated GLUT-4 transporter protein activity. This rapid phase window closes after approximately on hour. As such, CHO intake within this narrow timeframe is paramount to rapid glycogen repletion. Conversely, the second phase of glycogen synthesis is facilitated by insulin-stimulated GLUT-4 transporter protein activity. Glycogen resynthesis during this phase occurs more slowly.
There is evidence for a dose-response relationship between postexercise dosage of CHO ingestion and the rate of muscle glycogen resynthesis. For example, it has been shown that consuming 1.2 grams per kilogram per hour (g/kg/hr) CHO increased muscle glycogen content 150 percent more than 0.8 g/kg/hr CHO. However, ingestion of 1.6 g/kg/hr CHO provided no further increase in muscle glycogen content. Additionally, it was found that consuming 1.2 g/kg/hr CHO in smaller doses over a few hours is more effective at replenishing muscle glycogen than consuming one or two large doses. In summary, to optimize your clients’ muscle glycogen repletion, consume 1.2 g/kg/hr CHO at 15- to 30-minute intervals immediately following the end of the exercise session (Beelen et al., 2010). Table 1 offers practical recommendations for how your clients can meet this recommendation.
Table 1 |
Brigid (65 kg) is an endurance enthusiast and regularly completes moderate-to-vigorous workouts lasting longer than one hour. Her target CHO intake for the first two hours postexercise is approximately 160 g (65 kg x 1.2 g/kg/hr CHO x 2 hours). This sample menu of beverages and foods can be consumed every 30 minutes to achieve her CHO goal. |
Immediately postexercise |
8-ounce sports drink + 1 medium banana |
42 g CHO |
30 minutes postexercise |
8-ounce sports drink + 1 medium bagel |
53 g CHO |
1 hour postexercise |
1 cup yogurt |
42 g CHO |
90 minutes postexercise |
1 cup chocolate milk (low-fat) |
26 g CHO |
Total 163 g CHO |
Consuming protein along with CHO after exercise can accelerate the recovery process in two distinct areas. First, as previously highlighted, sufficient CHO intake facilitates muscle glycogen replenishment. Combining protein with CHO can attenuate this important physiological process, most notably when individuals have a difficult time ingesting the recommended 1.2 g/kg/hr CHO. Second, postexercise recovery also involves repair to muscle damage sustained during exercise training and skeletal muscle reconditioning. Favorable muscle adaptations (e.g., muscle hypertrophy) and repair to exercise-induced muscle damage are dependent on positive muscle protein balance. This scenario is only achieved with sufficient protein intake.
A number of studies have demonstrated that the combination of protein and CHO is superior to CHO alone at stimulating postexercise muscle glycogen synthesis (Beelen et al., 2010; Howarth et al., 2009). Specifically, consuming 0.4 g/kg/hr of protein and approximately 1.0 g/kg/hr CHO at 15- to 30-minute intervals can promote optimal muscle glycogen repletion (Table 2). However, there remains considerable debate in the scientific literature on the precise type of protein required to maximize postexercise muscle protein synthesis, although milk proteins and their isolated forms of whey and casein have proven to be effective (Beelen et al., 2010).
Table 2 |
Following is a sample menu that will make it possible for Brigid to meet target goals for CHO and protein intake to optimize postexercise glycogen replenishment and maintain a positive protein balance. These recommendations are based on current research findings. Her target CHO intake for the first two hours postexercise is approximately 130 g (65 kg x 1.0 g/kg/hr CHO x 2 hours). Her target protein intake for the first two hours postexercise is approximately 26 g (65 kg x 0.4 g/kg/hr protein x 2 hours). |
Immediately postexercise |
8 ounces water + 1 small banana |
23 g CHO |
1.0 g protein |
30 minutes postexercise |
8 ounces water + 1 medium bagel |
38 g CHO |
5.0 g protein |
1 hour postexercise |
1 cup vanilla yogurt + 1 ounce cashews |
43 g CHO |
16.0 g protein |
90 minutes postexercise |
1 cup chocolate milk (low-fat) |
26 g CHO |
5.0 g protein
|
Total 130 g CHO 30.0 g protein
|
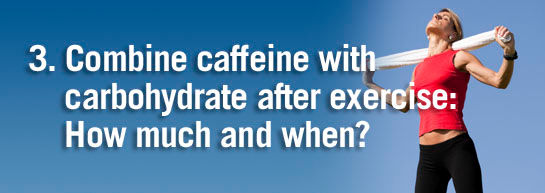
An additional strategy that can promote increased postexercise muscle glycogen synthesis is to combine caffeine with CHO intake. Two recent studies have shown this approach to be successful. In the first study, researchers reported that coingestion of 2 mg/kg/hr caffeine with 1.0 g/kg/hr CHO resulted in a 66 percent greater increase in muscle-glycogen synthesis rates over four hours of postexercise recovery (Pedersen et al., 2008). A separate study showed combined CHO and caffeine intake resulted in improved high-intensity interval-running capacity (Taylor et al., 2011). Participants initially completed a maximal exercise test to exhaustion. Throughout the four-hour recovery period, participants ingested 8 mg/kg/hr caffeine combined with 1.2 g/kg/hr CHO. Subsequent high-intensity interval-running performance was enhanced with this nutritional intake strategy compared to a condition where a similar CHO intake (i.e., 1.2 g/kg/hr) was combined with water.
While the mechanisms by which CHO and caffeine ingestion accelerate muscle glycogen synthesis are not yet fully understood, it is apparent that the combination of caffeine and CHO is an effective method of enhancing muscle protein resynthesis. For Brigid, the exerciser from the earlier example, the recommended 130 mg of caffeine postexercise could be achieved by drinking two 8-ounce cups of black coffee or tea over the initial two-hour postexercise recovery period.

As highlighted in the training-recovery article, recovery from cellular acidosis is essential for restoring the capacity to regenerate ATP from both the phosphagen system and glycolysis. While increased muscle-buffering capacity can be achieved through appropriate training, it can also be augmented with nutritional strategies. Alkalizing agents have been studied extensively for their potential to enhance performance by reducing the extent to which metabolic acidosis contributes to fatigue during high-intensity exercise performance (Peart, Siegler and Vince, 2012).
Sodium bicarbonate, in particular, has been found to improve recovery by promoting the removal of protons from skeletal muscle. Given the fact that increased concentrations of proton molecules within the muscle cell are detrimental to skeletal muscle performance, speeding up the rate of removal from the skeletal muscle environment will naturally result in a more rapid recovery. This, in turn, permits a better performance of subsequent high-intensity exercise bouts. The main drawback to using sodium bicarbonate is that some individuals experience gastrointestinal distress with its ingestion. As a result, it has been recommended that individuals purposefully experiment with the sodium bicarbonate–loading protocols to maximize the alkalizing effects and minimize the risk of potential symptoms. The recommended dosage and timeframe for sodium bicarbonate ingestion is 0.20.4 g/kg with 1 liter of fluids, one to two hours preexercise (Peart, Siegler and Vince, 2012). Sodium bicarbonate can either be ingested in capsule form or in a flavored beverage such as water. Brigid, for example, could mix 13 grams (65 kg x 0.2 g/kg) of baking soda with 1 liter of water with powdered water flavoring added.
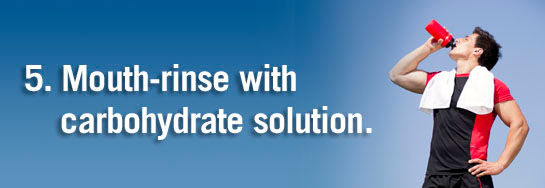
As discussed previously, repeated and prolonged high-intensity exercise can deplete muscle glycogen stores. Replenishment of muscle glycogen is critical to training recovery. Earlier we identified ingestion of CHO immediately postexercise as the most important strategy for promoting muscle glycogen synthesis. Yet during specific types of exercise (e.g., advanced resistance-training regimens) or sporting activities (e.g., soccer or hockey matches) the actual arrival of "postexercise" maybe not come for several hours. If a high level of performance is to persist throughout the later stages of these activities, some degree of nutritional recovery may be required throughout the activity itself to spare muscle glycogen. Undoubtedly, ingestion of CHO during exercise is a viable option. However, many individuals avoid consuming CHO-electrolyte beverages and/or CHO meals during exercise or competition due to the risk of gastrointestinal distress. Recent research has shown this dilemma may be avoided by simply mouth rinsing with a CHO solution without actually swallowing it (Rollo and Williams, 2011).
A number of studies have reported a 35% improvement in performance when mouth rinsing with CHO solution was performed throughout an extended bout of exercise (Rollo and Williams, 2011). The ergogenic mechanism behind this practice is a central effect. This is in contrast to the metabolic effect experienced when CHO is ingested. Research has shown CHO in the mouth stimulates reward centers in the brain, which subsequently results in increased motor excitability.
Individuals can mouth rinse with a small volume of liquid (i.e., 25 mL) with a CHO solution (~6%, which is comparable to most popular sports drinks) for five to 10 seconds throughout prolonged bouts of exercise (Rollo and Williams, 2011). This practice should be performed at quarterly intervals throughout the session. For instance, if the bout of exercise or activity lasts two hours, mouth rinsing can be done every 30 minutes.
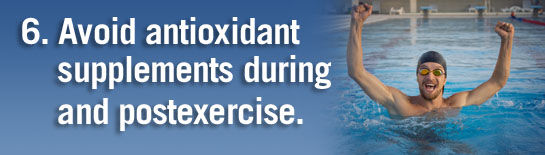
Free radicals, also commonly known as reactive oxygen species (ROS), are molecules that contain one or more unpaired electrons in their outer orbit. ROS are unstable in this state and, in an effort to become more stable, can react with other molecules. Therefore, ROS are harmful in that they can damage the functional capacity of these other molecules, such as cell membranes, DNA and proteins. In fact, ROS has been linked with cardiovascular disease, various forms of cancer and aging. Moreover, it is also recognized that ROS production occurs from numerous metabolic processes, including exercise. Because of the link between cell damage and ROS, conventional wisdom has been that antioxidant supplementation may benefit exercise performance and enhance recovery by countering the increase in ROS associated with exercise.
More recent research, however, is questioning the efficacy of this strategy. Over the past several years, studies have shown that the increase in ROS from exercise serves as an important signaling molecule, which ultimately results in important physiological adaptations.
In 2009, Ristow and colleagues showed that exercise-induced ROS production improved insulin resistance and caused a beneficial adaptive response promoting natural antioxidant defense capacity. They also noted that blocking exercise-dependent formation of ROS due to ingestion of antioxidant supplements eliminated the health-promoting effects of exercise. Simply put, exercise failed to elicit improved insulin sensitivity (Ristow et al., 2009).
Purposeful tactics are required to ensure adequate recovery from training. The strategies presented in this article are specific to recovery and should be integrated within, not substituted for, an overall healthy eating plan.
More recently, it has been shown that a transient increase in ROS stimulates mitochondrial biogenesis (Powers et al., 2010). Given the importance of healthy mitochondrial function to overall cardiorespiratory fitness and cardiovascular health, it appears unwise to immediately counter exercise-induced ROS production with supplemental antioxidants.
Therefore, while antioxidants are an essential component of the daily dietary intake, exercising individuals should avoid employing antioxidant supplementation as a strategy to promote recovery from exercise due to the fact that exercise-induced ROS mediates a number of the key health benefits accrued from exercise training.
References
Beelen, M. et al. (2010). Nutritional strategies to promote postexercise recovery. International Journal of Sport Nutrition and Exercise Metabolism, 20, 6, 515–532.
Howarth, K.R. et al. (2009). Coingestion of protein with carbohydrate during recovery from endurance exercise stimulates skeletal muscle protein synthesis in humans. Journal of Applied Physiology, 106, 4, 1394–1402.
Ivy, J.L. (2004). Regulation of muscle glycogen repletion, muscle protein synthesis and repair following exercise. Journal of Sports Science and Medicine, 3, 3, 131–138.
Peart, D.J., Siegler, J.C. and Vince, R.V. (2012). Practical recommendations for coaches and athletes: A meta-analysis of sodium bicarbonate use for athletic performance. Journal of Strength and Conditioning Research, 26, 7, 1975–1983.
Pedersen, D.J. et al. (2008). High rates of muscle glycogen resynthesis after exhaustive exercise when carbohydrate is coingested with caffeine. Journal of Applied Physiology, 105, 1, 713.
Powers, S.K. et al. (2010). Reactive oxygen species are signaling molecules for skeletal muscle adaptation. Experimental Physiology, 95, 1, 19.
Ristow, M. et al. (2009). Antioxidants prevent health-promoting effects of physical exercise in humans. Proceedings of the National Academy of Sciences of the United States of America, 106, 21, 8665–8670.
Rollo, I. and Williams, C. (2011). Effect of mouth-rinsing carbohydrate solutions on endurance performance. Sports Medicine, 41, 6, 449–461.
Taylor, C. et al. (2011). The effect of adding caffeine to postexercise carbohydrate feeding on subsequent high-intensity interval-running capacity compared with carbohydrate alone. International Journal of Sports Nutrition and Exercise Metabolism, 21, 5, 410–416.
__________________________________________________________________
Lance C. Dalleck, Ph.D., is academic coordinator of the cardiac rehabilitation postgraduate program at the University of Auckland in New Zealand. His research interests include improving exercise performance and health outcomes through evidence-based practice, quantifying the energy expenditure of outdoor and non-traditional types of physical activity, and studying historical perspectives in health, fitness and exercise physiology.