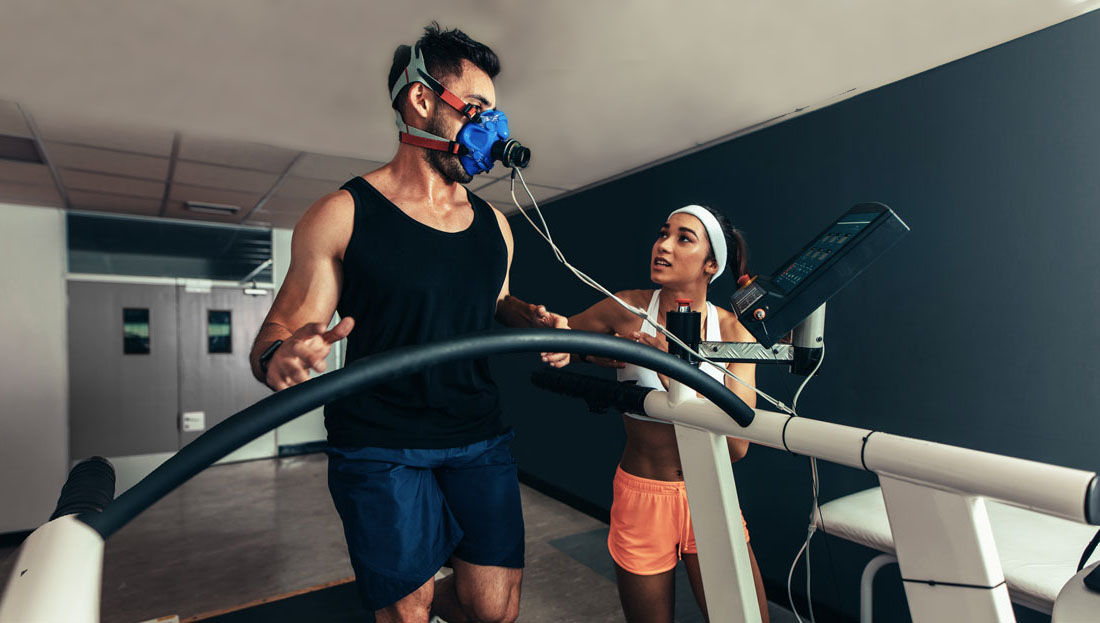
Isaiah A. St. Pierre, Christina A. Buchanan, Ph.D., and Lance C. Dalleck, Ph.D., with Daniel J. Green
Recovery is perhaps the most commonly overlooked aspect of people’s exercise regimens. Perhaps it’s because most people feel they’re not working hard enough at getting fit, so the need for rest seems counterintuitive. They’re more concerned about undertraining than overtraining. Or, perhaps it’s because it’s the least sexy aspect of training. After all, when’s the last time you saw a magazine cover with a hard-bodied fitness model touting the benefits of proper recovery?
The truth is, recovery from exercise training is a vital component of the overall exercise program, and is essential for optimal performance and continued progression. If the rate of recovery is appropriate, higher training volumes and intensities are possible without the detrimental effects of overtraining, which can include an increased resting heart rate, disturbed sleep, or decreased hunger on multiple days.
With that in mind, we sought to learn more about the best recovery strategy for optimizing the benefits derived from a training program. ACE enlisted the help of Lance C. Dalleck, Ph.D., and his team of researchers in the High Altitude Exercise Physiology Program at Western State Colorado University to investigate the effects of recovery on performance. The purpose of the study was threefold: (1) to compare the effect of active vs. passive recovery on endurance-related performance, (2) to compare the effect of active vs. passive recovery on power-related performance and (3) to compare the effect of different intensities of active recovery on exercise performance.
The Study
Fifteen participants were recruited to take part in this study. All of them were regular exercisers and were considered low-to-moderate risk. Their descriptive attributes were as follows:
- Age: 26.5 ± 8.4 years
- Weight: 154.9 ± 24.4 lb (70.4 ± 11.1 kg)
- Height: 67.6 ± 3.8 inches (173.4 ± 9.7 cm)
- Maximal oxygen uptake (VO2max): 50.2 ± 7.0 mL/kg/min
Three distinct studies were conducted. In all cases, the exercise trials were separated by 48 to 96 hours to prevent fatigue or a carryover effect.
Study 1: Effect of Active vs. Passive Recovery on Endurance-related Performance
To compare the effect of active vs. passive recovery on endurance-related performance, researchers had the participants perform two trials, each of which consisted of two running time trials to fatigue. For both trials, the participants exercised at 70% of their second ventilatory threshold (VT2) during the running bouts. Note: VT2 is a metabolic marker that represents the point above which high-intensity exercise can only be sustained for a brief interval due to an accumulation of lactate.
In one trial, participants performed a 15-minute active recovery consisting of a slow jog at 50% of their VO2max, then rested for an additional 45 minutes. In the other trial, the participants simply rested for the 60 minutes. In both trials, participants completed a second running bout at 70% of their VT2.
Blood lactate measurements were obtained at baseline, following the first maximal exercise test, throughout the recovery period and after the second maximal exercise test.
Study 2: Effect of Active vs. Passive Recovery on Power-related Performance
To compare the effects of the two types of recovery on power-related performance, the researchers again had the participants perform two trials, each consisting of two Wingate bouts performed in random order. The Wingate test is performed on a cycle ergometer and quantifies anaerobic power.
Between the two Wingate bouts, participants either performed a 15-minute active recovery consisting of slowly cycling at 50% of VO2max or did a passive recovery. Both groups then rested for an additional 15 minutes before the second round of exercise.
Study 3: Effect of Intensity of Active Recovery on Lactate Clearance and Endurance Performance
In this study, the participants completed three trials. Each trial consisted of a maximal exercise test on a treadmill and a supramaximal exercise bout (i.e., peak treadmill workout +5%), performed to fatigue. In between, the participants completed a 20-minute recovery at one of three active recovery intensities (80% of VT2; 90% of VT2; equal to VT2).
Blood lactate measurements were obtained at baseline, after the maximal exercise test, throughout the recovery period and after the supramaximal exercise bout.
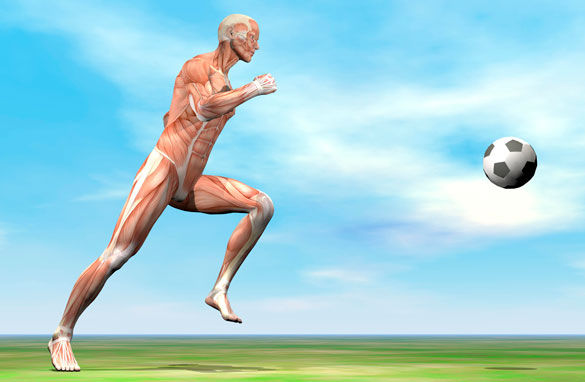
The Relationships Among Energy Pathways, Performance and Active Recovery
Skeletal muscle performance, be it sprinting down the field during a soccer match or cycling 40 kilometers in the middle stage of a triathlon, requires a constant supply of energy. The molecule adenosine triphosphate (ATP) provides the immediate source of energy for skeletal muscle contraction for these types of exercise scenarios. Given that ATP is essential for repeated muscle contraction, it would seem logical that large stores of ATP exist within skeletal muscle. This is not the case. In fact, if ATP could not be rapidly regenerated, the resting stores of ATP would quickly be depleted during high-intensity exercise scenarios.
There are three main energy pathways available to regenerate ATP—the phosphagen system, glycolysis and mitochondrial respiration. These pathways differ considerably in the maximal rate of ATP regeneration, and the duration for which this maximal rate can be sustained. Peak performance of the different energy systems can be compromised following challenging exercise conditions, such as repeated high-intensity sprints or extended submaximal running. Optimal recovery entails restoring the capacity for each energy system to function once again at maximal levels. Findings from the present study and elsewhere demonstrate that an active recovery is superior to passive recovery (Del Coso et al., 2010). Logically, from a physiological perspective, this should make sense. Continued blood flow to the skeletal muscle bed promotes the resynthesis of creatine phosphate and muscle glycogen stores, and simultaneously facilitates the removal of protons and lactate. Collectively, these factors aid in recovery.
The Results
Study 1: Effect of Active vs. Passive Recovery on Endurance-related Performance
When passive recovery took place between running bouts, the time to fatigue dropped by about 52 seconds in the second trial. When participants used active recovery, time to fatigue dropped by only 18 seconds from the first bout to the next, which is a statistically significant finding.
One goal of recovery is reducing blood lactate concentrations after exercise. As explained by Dr. Dalleck, the removal of lactate from the blood means that the lactate is instead being used by the heart and skeletal muscles.
As you can see in Table 1, the peak lactate values dropped significantly more quickly following active recovery.
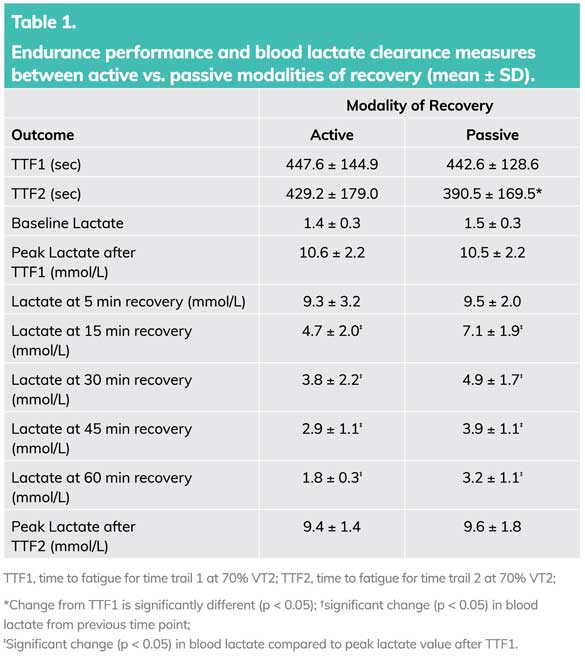
Study 2: Effect of Active vs. Passive Recovery on Power-related Performance
Peak power output and average power were preserved across the trials when participants underwent active recovery (Table 2). In contrast, passive recovery led to greater decrements in power from trial 1 to trial 2.
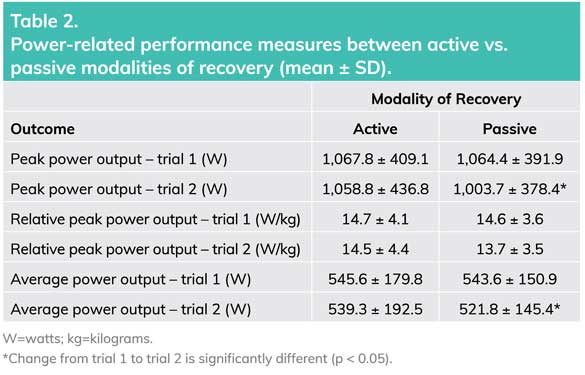
Study 3: Effect of Intensity of Active Recovery on Lactate Clearance and Endurance Performance
Active recovery performed at either 80 or 90% of VT2 elicited a greater peak workload during the supramaximal trial than did the more intense active recovery, which was conducted at an intensity equal to VT2. Blood lactate clearance was more rapid at the more moderate intensities as well (Table 3). In fact, after 20 minutes of active recovery at either 80 or 90% of VT2, blood lactate had returned to near baseline levels, but were still markedly elevated after 20 minutes of recovery at VT2.
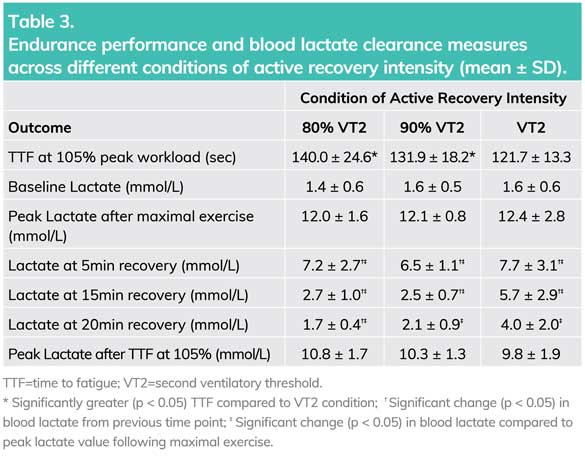
The Bottom Line
The research team offers three key take-home messages based on the findings of these studies:
- Active recovery was more effective than passive recovery at maintaining endurance performance. Indeed, endurance performance decreased between trials nearly three times more with a passive recovery (–11.8%) when compared to an active recovery (–4.1%).
- Active recovery was superior to passive recovery for sustaining power output. Impressively, in a second Wingate test, peak power output was virtually maintained (–0.8%) with an active recovery. In contrast, peak power output declined seven times more (–5.7%) with a passive recovery when compared to an active recovery.
- Active recovery should be performed at a moderate intensity (i.e., 80 to 90% of VT2, which is approximately 55 to 60% of heart-rate reserve) in order to sustain endurance performance and promote greater blood lactate removal. In contrast, when active recovery is performed at a vigorous intensity (i.e., at VT2, which corresponds to 75 to 85% of heart-rate reserve), there is delayed blood lactate removal and a resultant decrease in endurance performance.
Stated simply, recovery does not just take care of itself. It should be a planned element of any training program, particularly for athletes who are preparing for competition. For some people, Dr. Dalleck explains, “There may be more to be gained by focusing on recovery and making adjustments in that area than can be gained by modifying the training program itself.”
In other words, if a client is not making the gains you’d anticipated, lack of adequate recovery, or the wrong type of recovery, may be to blame. Coaches and trainers tend to be a bit over-ambitious and feel the need to push harder to drive results, especially if a particular client has that same mentality. It may be wise to instead take a step back to consider how a client is recovering between, and within, bouts of exercise, and then incorporate recovery as part of the client’s program.
Reference
Del Coso J. et al. (2010). Restoration of blood pH between repeated bouts of high-intensity exercise: Effects of various active-recovery protocols. European Journal of Applied Physiology, 108, 523-532.