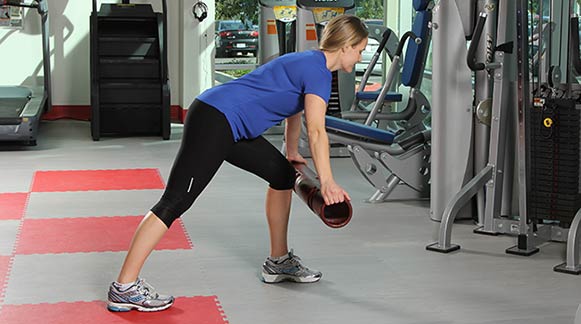
Among the most common reasons people cite for working with a personal trainer are to improve muscle strength, increase muscle size, enhance definition or lose weight, all of which can be achieved with resistance training. Your challenge as a personal trainer, therefore, is to determine how to help your clients achieve these goals in the fastest, safest, most effective way possible.
Conventional resistance training exercises most often utilize selectorized weight machines that use a cam or pulley to control the path-of-motion of the weight, or free weights such as barbells or dumbbells. Weight machines are designed to place the greatest amount of resistance at the point which a specific muscle is capable of producing the highest magnitude of force during a concentric (shortening) muscle action. Traditional free-weight exercises feature curvilinear joint actions, which typically take place in a single plane of motion in a single plane of motion, where muscles work to generate force directly against the downward pull of gravity.
For years strength training with moderate-to-heavy loads for a limited number of repetitions has been the accepted method for improving muscle size and definition, technically referred to as hypertrophy. However, recent research indicates that using lighter loads while moving in a variety of directions at different velocities may be the most effective method for developing integrated strength throughout the entire myofascial network. Understanding how muscle and connective tissue function to produce movement can provide you with the ability to create a wide variety of exercises to help clients achieve their goals of strength and muscular definition.
The Role of Muscles
Muscles control two different types of forces moving through the body: compressive forces, which create muscle shortening, and tensile or strain forces, which result in muscles lengthening (Myers, 2009). The balance between these two forces means that as muscle tissue on one side of a joint is shortening, the tissue on the opposing side experiences tension and strain as it is lengthened. While most traditional exercise machines focus on creating compressive forces within a muscle, it is actually the lengthening of the elastic fascia and connective tissue as a result of tensile forces that is responsible for producing the mechanical energy necessary for movement (Myers, 2009; Verkoshansky and Siff, 2006).
Customary resistance-training exercises focus on using the contractile element of actin and myosin myofilaments to generate concentric forces. However, during upright, functional movements it is the non-contractile, elastic component of the fascia and connective tissue responsible for generating the forces that produce movement. During dynamic movements, a muscle actually maintains an isometric contraction, where the fibers of the contractile element shorten to create tension in the elastic component as it stores mechanical energy during lengthening. This energy is then released when the tissue returns to its normal resting length (Schleip et al., 2012; Schleip and Muller, 2012; Myers, 2011; Myers 2009; Verkoshansky and Siff 2006).
Consider the example of multiple linear jumps: As an individual lands, the contractile element of the calves, thighs and hips shorten to place tension on the surrounding fascia and connective tissue as it is rapidly lengthened. As the tissue rapidly returns to its resting length, it can generate the forces to propel the individual forward into the next jump. This action is repeated during most movements, from walking to explosive weightlifting; the faster a tissue can lengthen, the quicker and more forcefully it can produce energy as it shortens (Schleip et al., 2012; Verkoshansky and Siff, 2006).
Many overuse injuries occur within the elastic component of fascia and connective tissue, which envelopes muscle tissue, from individual fibers (myofibrils) to bundles of fibers (the fascicles which comprise a muscle unit), when it is loaded beyond its capacity (Schleip and Muller, 2012; Myers, 2011). If the elastic tissue experiences tensile forces that exceed its functional capacity, it could create inflammation, which then limits the ability of the different muscle layers to slide against one another, thus restricting joint motion (Hamill et al., 2012; Myers, 2009).
The Emergence of a New Exercise Technique
Instead of conventional exercises, which focus on contraction of the non-elastic contractile element at one particular joint or muscle group, exercise programs that use a variety of loads while moving in a number of different directions at different speeds may be more beneficial for training the entire myofascial network as one integrated system (Schleip et al., 2012; Schleip and Muller 2012; Davids et al., 2003). Traditional resistance-training exercises and weight machines do not take advantage of the inherent elastic actions to create muscle force. This emphasis on strengthening the contractile rather than the elastic function of muscle could lead to potential injuries when an individual has to perform rapid movements or changes of speed (Schleip, 2012; Hamill et al., 2012). Think of a traditional bodybuilder who trains one joint and muscle group at a time on fixed-path-of-motion equipment. The bodybuilder can develop strength and increased cross-width of the contractile elements, but due to a lack of training, the elastic tissue can be susceptible to injury if he or she is called upon to move explosively or execute an unfamiliar movement pattern.
An emerging trend known as loaded movement training is an effective way to help clients develop total-body strength and muscle definition by training all of the muscles together as one system. Fitness educator and Institute of Motion founder Michol Dalcourt defines loaded movement training as “movement-based resistance training that combines full-body, task-oriented movement patterns with load.” Dalcourt explains that exercises can be arranged in to four general categories: Classic Resistance Training, Unloaded Linear Training, Unloaded Multi-planar Training and Loaded Movement Training. Table 1 describes each general category (Dalcourt, 2013).
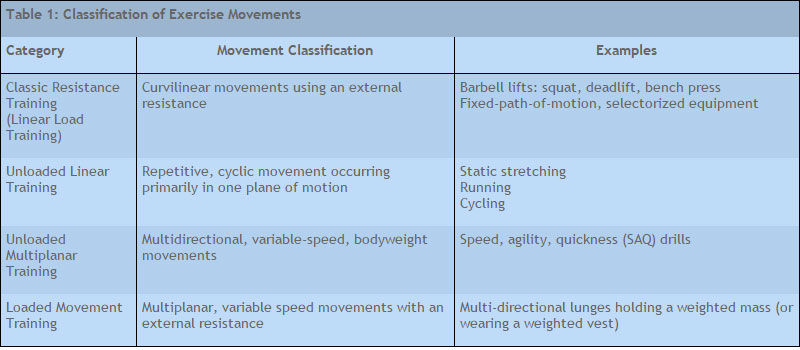
Benefits of Loaded Movement Training
According to Dalcourt, the benefits of loaded movement training include (Dalcourt 2013):
- Integration of a number of physiological systems: muscle, fascia and connective tissue, nervous and skin. (The largest organ in the body, skin is very elastic and multidirectional exercises can help improve its elasticity and appearance.)
- Lower compressive forces on the joints and skeletal structures of the body; traditional resistance training focuses on lifting heavy loads directly against gravity while loaded movement training focuses on movements through gravity, which increases tension on the fascia rather than compressive loading of the joints.
- Using a variety of loads combined with different starting positions and movement patterns can improve multidirectional stability, mobility, strength and power.
This concept of loaded movement training is supported by a wide variety of evidence from research literature. When writing about exercise strategies for optimal function of muscle fascia and connective tissue, Schleip notes that “if one’s fascial body is optimally elastic and resilient, then it can be relied upon to perform effectively while offering a high degree of injury prevention” (Schleip and Muller, 2012). The right type of exercise program can enhance the elasticity and structural integrity of fascia, and proper loading through exercise can influence a younger, more youthful architecture of connective tissue (Schleip et al., 2012; Myers 2011; Myers, 2009). Multidirectional and multi-speed exercises for the entire fascial network can actually reduce the effects of aging and help produce more elastic tissue that is more youthful in architecture and function (Schleip et al., 2012; Schleip and Muller, 2012; Myers, 2009).
Real-life Situations
When training hockey players at the start of preseason in Canada, Dalcourt noticed that the players who spent their off-seasons working on their family’s farm were often much stronger than the players who spent their time training in the weight room. As Dalcourt investigated this phenomena he realized that most activities of daily living (ADLs) and athletic movements outside of the training environment require an indvidual to move a mass through gravity as opposed to lifting a mass vertically against it (Dalcourt, 2013).
For example, the barbell squat requires an individual to set his or her feet in parallel and maintain spinal extension and a symmetrical alignment in the hips, while lowering and raising the barbell against gravity. Compare that to a woman spending the day working in her garden; as she’s digging in the dirt she will be performing a variety of different squats with her feet and hips in a variety of different positions. Doing perfectly symmetrical squats in the gym will help improve strength in a specific pattern with an emphasis on concentric muscle action, but it won’t adequately develop her ability to use her fascia to produce and mitigate forces across the entire network, which is what actually happens as she’s going about her chores.
In another example, consider how most parents pick up their kids off of the ground; they don’t take the time to set their feet in a neutral position, position their perfectly still child symmetrically position relative to his or her midline and then, after bracing, symmetrically load each arm as they pull the child to their chest. As most parents will tell you, there is no cognitive thought relative to their body position as they perform the movement of picking up their child. They simply hold on to their child however they can and lift them to whatever position is manageable at the time. These two examples briefly demonstrate that, whether in sports or activities of daily living (ADLs), the only constant in human movement is continuous variability.
The Role of Movement Variability
Researchers are beginning to understand the role that variability plays in optimal movement efficiency and why loaded movement training is such an important concept to consider when developing an individual’s exercise program (Hamill, Palmer and Van Emmerik, 2012; Timmons, 2010; Stergiou, Harbourne and Cavanaugh, 2006). Loaded movement training can be used to apply constant variability by manipulating the direction, distance or speed of an exercise. A client who can perform lightly loaded resistance-training exercises in a variety of movements patterns can train and prepare for the constant changes in direction and velocity that occur during dynamic movement.
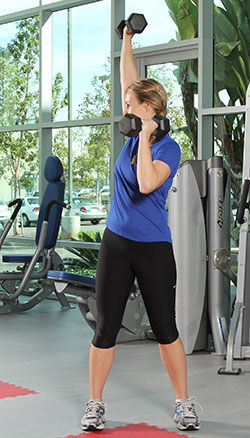
This concept applies to athletes as well; the trend of the past number of years is to train athletes in “functional” movement patterns specific to the demands of their sport. Regardless of what sport an athlete is preparing for, the likelihood that they will perform exactly the same movements exactly the same way in every practice or competitive situation is extremely small. During competition, athletes have to constantly adapt their body movements to the locations of both their teammates and opponents. Research indicates that high-performing athletes depend on constant movement variability to achieve success. Time-motion analysis studies indicate that even at the highest levels of performance, many athletes do not perform successive movements exactly the same way. In fact, the highest-level performers actually demonstrate a wide variance in movement patterns when executing a play (Hamill, Palmer and Van Emmerik, 2012; Barlett, Wheat and Robins, 2007; Bartlett, 2008; Stergiou, Harbourne and Cavanaugh, 2006). Loaded movement training allows athletes to develop sport-specific strength by using variable, high-velocity movements corresponding to game-like situations. This helps them to have a greater carryover effect from the conditioning room to the competition arena (Mohammed et al., 2012; Timmons, 2010).
For both athletic movements and ADLs, the imposed demand of "real-time" motion is a reactive, instinctive movement into "non-traditional" body positions as the individual adjusts to his or her environment. The result is a slightly different movement pattern with each subsequent movement demand. This is the paradox in the principle of specificity: The movement demands of sport and life are innately variable and often are unreplicable (Bartlett, Wheat and Robbins, 2007; Bartlett, 2008). If this were not true, every basketball player would achieve 100 percent accuracy with their free throws because they shoot from a static position without the distraction of opponents moving toward them as they set up for the shot. In addition, if team-sport (football, basketball, soccer, etc) athletes moved exactly the same way every play, it would be relatively easy to prepare strategies and techniques for defeating an opponent. Even though an athlete might have a scripted play, he or she will use minor changes in body position and movement to react to opponents during a competition.
Mechanical Stimulus for Muscular Development
Even though it is a good idea to use lighter loads when challenging clients to move in multiple directions, loaded movement training can provide an adequate stimulus to generate muscle hypertrophy. Mechanical tension, metabolic stress and subsequent damage to (and repair of) the actin and myosin protein filaments are the probable mechanisms of muscle growth (Schoenfeld, 2010). How does a lighter load create the levels of mechanical stress to stimulate muscle growth? To answer that question it is important to do a brief review of physics. Newton’s second law of physics defines force as a product of an object’s mass and its acceleration (F = MA). Traditional methods of training for hypertrophy have focused on the mass component by using heavier loads to generate greater muscle force (expressed as force = MASS x acceleration). In most cases, when an individual lifts a heavy load the amount of mass is so great that it restricts its acceleration, which limits the amount of force generated. Reducing the mass and increasing the acceleration of an object can allow an individual to generate a greater amount of muscle force during an exercise movement (force = mass x ACCELERATION). Loaded movement training can be used to challenge clients to move lighter loads in multiple directions at explosive speeds.
Hypertrophy is primarily the result of an increase in the crosswidth of type II muscle fibers. Type II muscle fibers are controlled by type II motor units, which require a high-threshold stimulus for activation. Classified as the Dynamic Effort method, lifting a non-maximal load at the highest attainable speed involves type II motor units and muscle fibers to improve the rate of force development and explosive strength (Zatsiorsky and Kraemer, 2006). Power is defined as the rate of doing work and is expressed by the equation Power = Work/Time (where Work = Force x Distance).
The purpose of strength training is to stimulate muscle growth by activating and fatiguing involved muscle fibers and motor units. Muscle motor units are recruited via the size principle, where smaller, type I motor units (and attached muscle fibers) are recruited before the larger type IIs responsible for muscle hypertrophy (and definition) (Zatsiorsky and Kraemer, 2006). This explains why heavy loading is used when training for hypertrophy. Unless repetitions are performed to momentary muscle failure, the use of lighter loads (lower intensity) moved at a steady tempo does not create the overload necessary to fatigue the type I motor units and muscle fibers. Therefore, this approach does not activate the type II motor units and muscle fibers that initiate muscle growth.
However, recent evidence indicates that moving a lighter load at a faster velocity can stimulate the larger type II motor units to generate the necessary muscle power. Mohamad and colleagues (2012) found that, when equated for total training volume, a lower intensity of resistance moved at a higher velocity can create an effective stimulus for a hypertophic training effect. The study authors found that while the heavier loading scheme of 70% 1RM allows for greater time under tension (TUT) and force output for a single repetition, it is important to consider the total output of a training session. The lighter load of 35% 1RM actually produced the higher values (Mohamad, Cronin and Nosaka, 2012). These findings suggest a potential shift in a principle tenet of resistance training, which held that only heavy loads can stimulate muscle growth. Instead, this research supports the idea that loaded movement training can be an effective method for improving strength and muscle size.
Another consideration is that the concentric action of the prime mover is only one half of the equation during a movement. Training with lighter loads for faster velocities requires the antagonistic groups of the involved muscles to rapidly lengthen, creating high eccentric loads as the agonist concentrically shortens. This rapid eccentric lengthening of involved fascia can create the damage to actin-myosin protein filaments to stimulate the process of protein synthesis for tissue repair. “From a hypertrophy standpoint," writes fitness expert Brad Schoenfield, "speed of movement may have a greater importance on the eccentric component of a repetition….eccentric actions have the greatest effect of muscle development. Specifically, lengthening exercise is associated with a more rapid rise in protein synthesis” (Schoenfeld, 2010).
Adding Loaded Movement Training to Your Clients' Programs
The idea that rapidly accelerating a light intensity allows an individual to increase the size and strength of the involved muscles has profound implications for developing a client’s exercise program. Lighter loads allow a greater variety of movements to create an ideal stimulus of involved fascia and connective tissue. Due to their length standard, Olympic barbells are not effective tools for loaded movement training; as a result of his research on how movement affects the human body, Dalcourt created the ViPR specifically for the purpose of loaded movement training. Other fitness equipment that can be used to add a load to multiplanar movement includes cable columns, medicine balls, small sandbags (or Sandbells™) and dumbbells.
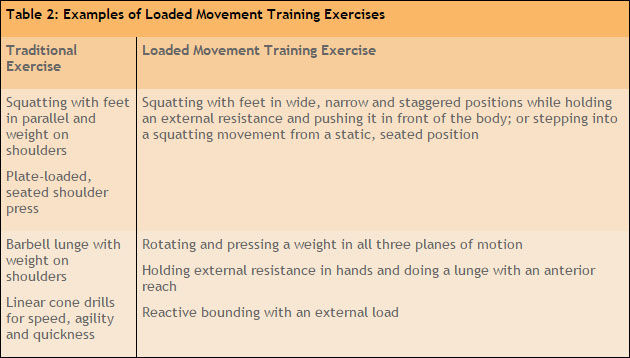
Summary
“What is clear is that the cellular mechanisms that regulate muscle hypertrophy are complex and work in a dynamic fashion” (Spangenburg, 2009). Using traditional resistance-training exercises with maximal loads remains the most effective method for improving strength. However, adding loaded movement training to a client’s exercise program will greatly improve his or her ability to execute a variety of tasks and movements they may be challenged to do during a daily routine or athletic event. Performing multidirectional movements while carrying loads like groceries, laundry or even young children is the kind of thing many clients do in their ADLs, but is often omitted from traditional exercise programs. The concept of loaded movement training as described by Dalcourt and supported by a wide variety of scientific literature should be considered a missing element in current exercise protocols, and personal trainers should think about adding it to their client’s workout programs. Understanding how to use the latest findings in science to apply loaded movement training with variability to clients' exercise programs can provide you with the safest, most effective solutions for producing total-body strength and muscular definition, which will help your clients reach their training goals in a time-efficient manner.
References
Bartlett, R., Wheat, J. and Robins, M. (2007). Is movement variability important for sports biomechanists? Sports Biomechanics, 6, 2, 224-243.
Dalcourt, M. (2013). Loaded movement training: A missing link in today’s training protocols.
Davids, K. et al. (2003). Movement systems as dynamical systems: The functional role of variability and its implications for sports medicine. Sports Medicine, 33, 4, 245-260.
Folland, J. and Williams, A. (2007). The adaptations to strength training. Sports Medicine, 37, 2, 145-168.
Glasgow, P., Bleakley, C. and Phillips, N. (2013). Being able to adapt to variable stimuli: The key driver in injury and illness prevention? British Journal of Sports Medicine, 47, 2, 64-65.
Hamill, J., Palmer, C. and Van Emmerik, R. (2012). Coordinative variability and overuse injury. Sports Medicine, Arthroscopy, Rehabilitation, Therapy and Technology, 45, 4, 1-9.
Mohamad, N.I., Cronin, J.B. and Nosaka, K.K. (2012). Difference in kinematics and kinetics between high- and low-velocity resistance loading equated by volume: Implications for hypertrophy training. Journal of Strength and Conditioning Research, 26, 1, 269-275.
Myers, T. (2009). Anatomy Trains (2nd ed.). London: Elsevier.
Myers, T. (2011). Fascial fitness: Training in the neuro-myofascial web. IDEA Fitness Journal, 38-45.
Schleip, R. et al. (2012). Fascia: The Tensional Network of the Human Body. London: Elsevier.
Schleip, R. and Muller, D.G. (2013). Training principles for fascial connective tissues: Scientific foundation and suggested practical applications. Journal of Bodywork and Movement Therapies, 17, 103-115.
Schoenfeld, B. (2010). The mechanisms of muscle hypertrophy and their application to
resistance training. The Journal of Strength and Conditioning Research, 24, 10, 2857-2872.
Spangenburg, E.(2009). Changes in muscle mass with mechanical load: Possible cellular
mechanisms. Applied Physiology, Nutrition and Metabolism, 34, 328-335.
Stergiou, N, Harbourne, R. and Cavanaugh, J. (2006). Optimal movement variability: A new theoretical perspective for neurologic physical therapy. Journal of Neurologic Physical Therapy, 30, 3, 120-129
Timmons, J. (2011). Variability in training-induced skeletal muscle adaptation. Journal of Applied Physiology, 110, 846-853.
Verkoshansky, Y., and Siff, M. (2006). Supertraining, 6th ed. Supertraining: Rome, Italy.
Zatsiorsky, V. and Kraemer, W. (2006). Science and Practice of Strength Training, 2nd ed. Human Kinetics: Champaign, Ill.