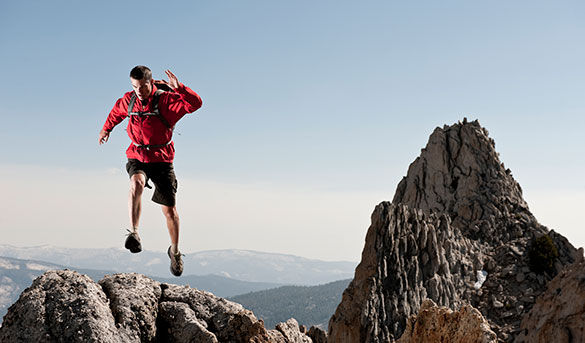
Athletes and researchers have long been fascinated with harnessing the power of training at high altitudes, as the hypoxic conditions increase red blood cell production, which in turn increases the oxygen-carrying capacity of the blood. An increase in oxygen-carrying capacity has been correlated to improvements in maximal oxygen uptake (VO2max) and endurance performance (Levine and Stray-Gundersen, 1997). Of course, finding an edge in the quest for improved endurance training is a Holy Grail of sorts for researchers, coaches and elite athletes around the world.
One of the limiting factors in the early research on altitude training was that athletes weren’t always able to exercise at the same intensity once they moved to a higher elevation (Levine and Stray-Gundersen, 1997). The relative availability of oxygen in the air is reduced as one climbs in altitude, and because there is less pressure to drive the oxygen molecules into the blood as it passes through the lungs, the oxygen in the blood is reduced. Therefore, an athlete training at high altitude will not be able to deliver as much oxygen to working muscles, necessitating a reduction in exercise intensity to keep the heart rate in a target zone.
To compensate for this, researchers experimented with various combinations of living at a certain elevation while training at another (e.g., “live high-train low” or “live high-train low and high”) to try to find the key to eliciting the benefits of exercising in the thinner mountain air without adversely affecting training intensities.
Another tool used to stimulate increased exercise performance is inspiratory muscle training (IMT), a protocol of breathing exercises that aims to strengthen the respiratory muscles that is often used by people with asthma, bronchitis or emphysema. Romer, McConnell and Jones (2002) found that six weeks of IMT resulted in significant improvements in pulmonary and respiratory function and significant improvements in performance.
All of this information illustrates the complexity that researchers and manufacturers face in developing protocols and devices to mimic elevation training. Training Mask, LLC, the makers of the Elevation Training Mask 2.0 (ETM) believe they have done just that. The ETM covers the nose and mouth and has different-sized openings and flux valves that can be adjusted to simulate different elevations (from 3,000 to 18,000 feet, or 914 to 5,486 m) by making breathing more difficult.
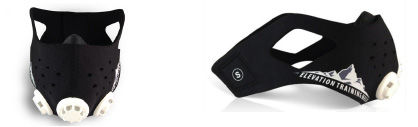
ACE enlisted the help of John Porcari, Ph.D., and his team of researchers in the Department of Exercise Sport Science at the University of Wisconsin–La Crosse to test the ETM, specifically the manufacturer’s suggestions that it can increase endurance and VO2max, as well as improve lung function.
Understanding the Nomenclature
The ACE textbooks use different terminology than is used in this research. What is called the ventilatory threshold, or VT, in this article is called the first ventilatory threshold (VT1) in the books, while the respiratory compensation threshold, or RCT, is called the second ventilatory threshold (VT2). The terminology can get a bit confusing, as there are various and sometimes-conflicting names related to these metabolic markers, which is why ACE chose to simplify their use throughout its textbooks. When reading this article, remember:
- VT = VT1, or the point at which ventilation deviates from a progressive linear increase. If an exerciser can talk comfortably in sentences that are more than a few words in length, he or she is likely below this metabolic marker.
- RCT = VT2, or the point at which lactic acid accumulates at faster rates than the body can buffer and remove it. This marker represents the highest sustainable level of exercise intensity, a strong indicator of exercise performance.
The Study
The purpose of this study was to determine the effect of training while wearing the ETM on ventilatory threshold (VT), respiratory compensation threshold (RCT), VO2max, power output, lung function and hematological variables.
Twenty-four moderately trained volunteers served as the study subjects, all of whom were students at the university. None of the participants were involved in any cycling training during the previous six months. Each subject first completed a maximal cycle ergometer test to determine his or her VO2max, VT, RCT, maximal heart rate (HR) and maximal power output. Pulmonary function was also assessed for each subject.
The participants were then divided into a “mask group” and a “control group” (Table 1). The groups completed identical training programs, the only difference being whether or not they wore the mask. After some preliminary sessions during which the participants grew familiar with the training program and the mask group got comfortable with the mask itself, a six-week high-intensity cycle ergometer-training program began.
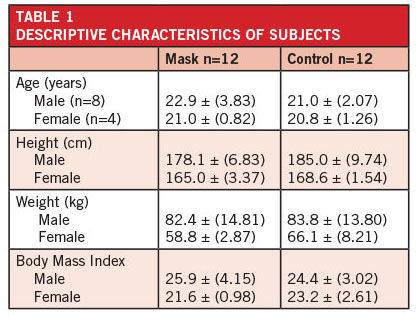
Training sessions were held twice a week and each session was 30 minutes long—a five-minute warm-up, 20 minutes of high-intensity intervals and a five-minute cool-down. The 20-minute interval segment included 10 repetitions of 30 seconds of work at the subject’s individualized peak power output, followed by a 90-second recovery period. Participants wore HR monitors and HRs were recorded at the end of the high-intensity portion of each interval. Ratings of perceived exertion (RPE) (0–10 scale) were also recorded at that time, along with session RPEs after each workout. Training intensities were adjusted throughout the six-week period based on subjects’ RPE after the tenth interval of each workout.
During week 1, the masks were set to simulate an altitude of 3,000 feet (914 m); during week 2, they were set to 6,000 feet (1,828 m); during weeks 3 and 4, they were set to 9,000 feet (2,743 m); and during weeks 5 and 6, they were set to 12,000 feet (3,658 m).
At the end of the training program, subjects in both the control and mask groups again completed the pre-study test battery.
The Results
There were no significant changes in lung function or hemoglobin or hematocrit levels for either group as a result of training. Both the mask and control groups saw significant increases in VO2max and peak power output as a result of training, but there was no difference in the magnitude of improvement between groups. However, the mask group had significant improvements in VT, power output at VT, RCT, and power output at RCT as a result of training (Table 2). It should be noted, however, that only the increases in RCT and power output at RCT were significantly greater than in the control group. The increases in VT and power output at VT were of a similar magnitude (about 15 percent) to the changes at RCT, but did not reach statistical significance.
To quantify the intensity of training across the duration of the study, the research team recorded exercise HR, session RPE and workload (in watts). There were no significant differences in exercise HR between the groups and it was consistent across the 12 sessions. Session RPE was also consistent across the 12 sessions, though it was significantly higher for the mask group (6.2 ± 0.74) than for the control group (5.5 ± 0.67) due to the increased resistance of breathing through the mask.
Things get a bit more interesting when looking at workload (Figure 1). While there was no significant difference in the average workload between the groups, the exercise workloads for the mask group were significantly greater during sessions 11 and 12.
Figure 1. Average workloads of the mask and control groups over the 12 training sessions
The Bottom Line
There were a lot of variables tested in this study—from RPE to hematocrit levels—again reminding us of the complexity of trying to mimic the power of elevation training. That said, there are important and clear results to discuss.
Let’s look back at the claims made by the manufacturer that wearing the Elevation Training Mask 2.0 could increase endurance and VO2max and improve lung function. The current study did not bear this out. While VO2max improved in the mask group over the course of the study, a similar improvement was seen in the control group. In addition, there was no change in lung function over the 12 weeks.
The name of the device and the settings it provides would make you believe that the mask simulates different levels of altitude training. However, the decreases in saturation of oxygen in the blood were small while wearing the mask (2 percent), which is far below the desaturation experienced when a person actually climbs to higher elevations. There is no doubt, however, that wearing the mask makes it more difficult to breathe, making it more like an inspiratory muscle training device.
While there were differences in the magnitude of the increase in VO2max and maximal power output between the mask and control groups, the mask group did have close to 15 percent increases in VT and RCT and the associated power output seen at both of those thresholds. The exact reason for those increases is unclear, but may be related to the trapping of carbon dioxide within the mask during exercise. Regardless of the mechanism, it is important to note that these improvements have significant performance implications.
“Remember,” says Dr. Porcari, “the ventilatory threshold is the primary limiting factor of endurance capacity, so making improvements there is potentially very exciting.”
Dr. Porcari states that a longer-term training protocol would be needed to see if the improvements seen in the lab can be translated to performance in the real world. If so, he says, “the ETM could be a very valuable training adjunct for endurance athletes who are looking to improve their performance.”
References
Levine, B.D. and Stray-Gunderson, J. (1997). “Living high-training low”: Effect of moderate-altitude acclimatization with low-altitude training on performance. Journal of Applied Physiology, 83, 1, 101–112.
Romer, L.M., McConnell, A.K. and Jones, D.A. (2002). Effects of inspiratory muscle training on time-trial performance in trained cyclists. Journal of Sports Sciences, 20, 547–562.